This is the fourth of a series of articles focusing on how semiconductor technologies can revolutionize proteomics.
We already gave an overview of the challenges and opportunities characterizing this domain, looked into how integrated sample prep can improve tools, and dove into the sensing part and how chip technology can contribute in making the tools and assays higher throughput, faster and more compact.
Want to stay informed about how semiconductor technologies revolutionize proteomics?
Nanopores are tiny holes, typically just a few nanometers in diameter, that can be used to detect, quantify and sequence single molecules such as DNA, RNA, and proteins. For this to work, they need to be combined with:
- ingenious fluidics to bring the single molecules to the holes
- a functionalized surface
- some electronics to capture the signal (e.g. a current or voltage change)
- and data processing to translate the signal into useful information.
Nanopore-based sensing is a label-free technique and requires no amplification steps which is for example beneficial when determining an exact viral load in a blood sample or parsing through a sample made up of proteins.
For DNA and RNA sequencing, there are already nanopore-based commercial applications on the market (from Oxford Nanopore). Sequencing via nanopores has the advantage of tackling long reads which translates into more reliable and faster results.
For proteomics, nanopores promise to be a key sensor technology for the third generation of techniques focusing on high-throughput single-molecule protein sequencing. Also for other applications, nanopores can be an interesting route to follow: e.g. to efficiently read out the DNA barcodes used for protein detection, to analyze proteoforms, to realize DNA memory applications, or to gain insights into the gut microbiome.
Nanopore arrays promise to be a key sensor technology for the third generation of proteomics techniques.
Turning to nature or to the semiconductor industry?
Biological nanopores – made of engineered proteins – are the ones used in today’s commercial applications. They offer a high specificity and selectivity but must deal with a limited lifespan and stability issues. They are embedded into membranes via a complex and time-consuming process.
Solid-state nanopores are mostly only made at lab scale, but as they can rely on the well-established manufacturing expertise of the semiconductor industry, they hold the promise of scalability, mass-producibility and easy integration with other functionalities such as readout electronics or microfluidics.
Hybrid nanopores – biological pores embedded in solid-state nanopores – combine the best of both worlds: the specificity of biological nanopores and the robustness of solid-state materials.
As aforementioned, the problem with all kinds of nanopores is their production, and their stability in wet environments. It’s often a complex (lab-scale) process, resulting in many companies leaving this promising technology for what it is, and very little applications appearing on the market. Despite the technology’s great promises.
A milestone: mass-manufacturable, stable nanopores
Imec has succeeded in establishing a process to mass produce solid-state nanopores in a membrane using deep UV lithography in its 200mm cleanroom. The approach is transferable to a 300mm cleanroom, and potentially scalable under 10nm. This is a huge leap forward as it will provide the sandbox onto which new applications can be build and is a required condition to do assay development.
The fact that there is extremely little variability in size makes the nanopores the ideal choice for hybrid nanopores. Indeed, when combining biological nanopores with solid state nanopores, the size must be very accurate to fit in the biopore.
Its mass producibility is significant in two ways. First, it paves the way for many application innovations since companies can now readily experiment with the technology for applications in personalized medicine, proteomics, etc.
Secondly, it enables gathering a lot of data on nanopore functioning, and the role of materials, process parameters, etc. Based on these data, models can be calculated which will result in more insights, learning, and eventually the manufacturing of better nanopores, tuned to specific applications.
Imec provides a manufacturable nanopore platform on which single-molecule sensors can be built. Imec is actively looking for assay partners that can bring in the necessary chemistry know-how. These partners can test their applications on the minimal viable demonstrator (MVD) that imec is currently developing: a multi-pore array combined with fluidics and read-out electronics.
Addressing the next hurdle: fluidics integration
With the above result, the first hurdle was tackled: making nanopores readily available, via an efficient and easy-to-scale process. However, a nanopore is a hole in a membrane and does not as such function as a single-molecule sensor. It needs some extra functionalities.
To that end, imec is developing the fluidics and electrical readout modules to produce an individually addressable array of solid-state nanopores. A minimal viable demonstrator will allow further application development.
Conclusion
Imec's aim to mass-produce stable and reliable solid-state nanopores is key for the field of single-molecule sensing. The ability to produce sub-5nm nanopores with uniform size and high stability in wet environments overcomes one of the biggest hurdles in nanopore technology. This advancement not only opens the door for extensive experimentation and application development, but also provides a wealth of data to refine and enhance nanopore designs further.
Moving forward, the challenge lies in integrating microfluidics to enable individually addressable nanopores in high-density nanopore arrays on chip. By addressing this, imec continues to push the boundaries of what is possible in nanopore technology, paving the way for new breakthroughs in personalized medicine, proteomics, and beyond.
More reading
- In our white paper ‘Monolithic microsystems’ you can find more information on imec’s post-processing and integration expertise, focusing for example on microfluidics, nanostructures, electrodes etc.
- Imec's Digiome project aims to realize the promise of nanopore protein sequencing through open innovation.
- Want to talk to us about your ideas and/or our expertise on health technologies? Please contact health@imec.be.
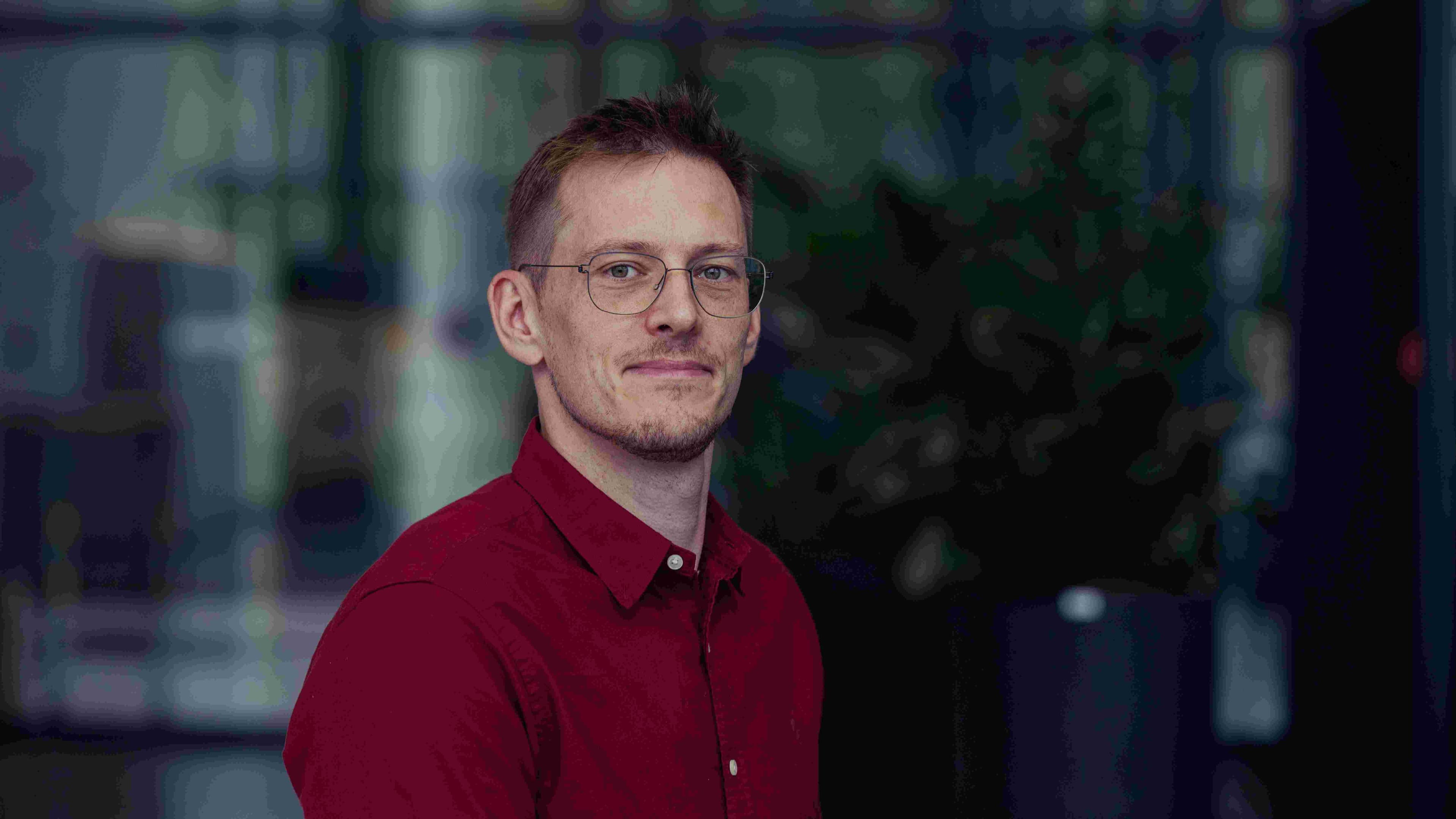
Sanjin Marion is a physicist and biophysicist specializing in nanotechnology, currently working at imec in Leuven, Belgium, where he serves as a Principal Member of Technical Staff. He completed his PhD in Physics in 2017, with a focus on nanofluidics and nanopore technologies. Marion’s research includes biophysics, nanotechnology, and molecular electronics, particularly in applications such as biomolecular sensing and nanopores for genomic analysis. He is recognized for his interdisciplinary work combining physics with biological systems.
More about these topics:
Published on:
22 October 2024