A very practical model
It was in May of last year that imec announced it had developed a simulation model. This model uses the weather conditions to predict how much energy a solar panel will ‘actually’ produce. Typically, a solar cell has a specific conversion efficiency that is expressed in %. This percentage is measured in a laboratory under standard conditions. But when this solar cell is used in a module or panel - whether it is installed on a roof or as part of a solar farm - and it has to operate in all sorts of weather conditions, the actual yield of the cell is sometimes very different. For the managers of solar farms, energy providers, developers and investors, etc. it is important to know how much power a solar panel, when installed in a particular location, will generate on an annual basis. For them, the most important parameter is not the conversion efficiency of the panel, but the euro per kilowatt hour it generates. And this is precisely where the simulation model can help them. Below, we have set out some of the applications for which the simulation model can be of value:
- Load management. To be able to guarantee the stability of the energy network, it is important for supply and demand to be balanced at all times. This can be difficult when renewable forms of energy – such as solar – are included in the network. This is because the amount they generate is very much dependent on the weather. Using imec’s new simulation model, it is possible to predict how much solar en will be produced – right down to a 15-minute window of accuracy. In the short term, the model can be used in this way for (micro-)grids, such as islands, large manufacturing facilities, residential districts – and so on.
- Negotiating energy prices. Energy is traded between countries. To negotiate from as great a position of strength as possible, it is important to know exactly how much energy your country will produce over a particular period of time. The actual amount of solar energy you are going to produce can be computed accurately using the simulation model. As a result, solar energy is becoming less and less of an ‘unpredictable’ factor in the supply of energy.
- Technical adjustments to the solar cell. The simulation model also makes it possible to calculate how much a specific change made to a solar cell will produce ‘in reality’. Imagine, for instance, that you want to use a different type of material in the cell, or that you want to replace the glass cover with film, etc. The model computes how much the altered cell would produce in the solar cell farm under weather conditions typical for the area where it is located. This enables PV researchers and solar cell manufacturers to make the right choices when optimizing a solar cell, module and panel. And they can do this without actually having to produce the cells, saving a great deal of time and money.
- Installing the solar panel. In countries such as Belgium, solar panels are typically positioned at an angle of 36° to achieve the right light level. But what if you were to place it at 30°? If you did that, you might have less light exposure, but you might also achieve more cooling by the wind – and hence enable the solar panels to function better. This could mean that it might generate more energy at 30° than at 36°. Optimizing scenarios such as this are straightforward enough with the new simulation model.
What makes this model so unique?
Imec’s new simulation model is holistic in that it takes optical, thermal and electrical parameters into account. It also looks at how these parameters interact with each other. For example: if more light hits the cell (optical parameter), this has an effect on how the cell heats up (thermal parameter) and also on the production of the charge carriers within the cell (electrical parameter). Which means there are lots of scenarios in which this interaction of parameters is able to produce the end result.
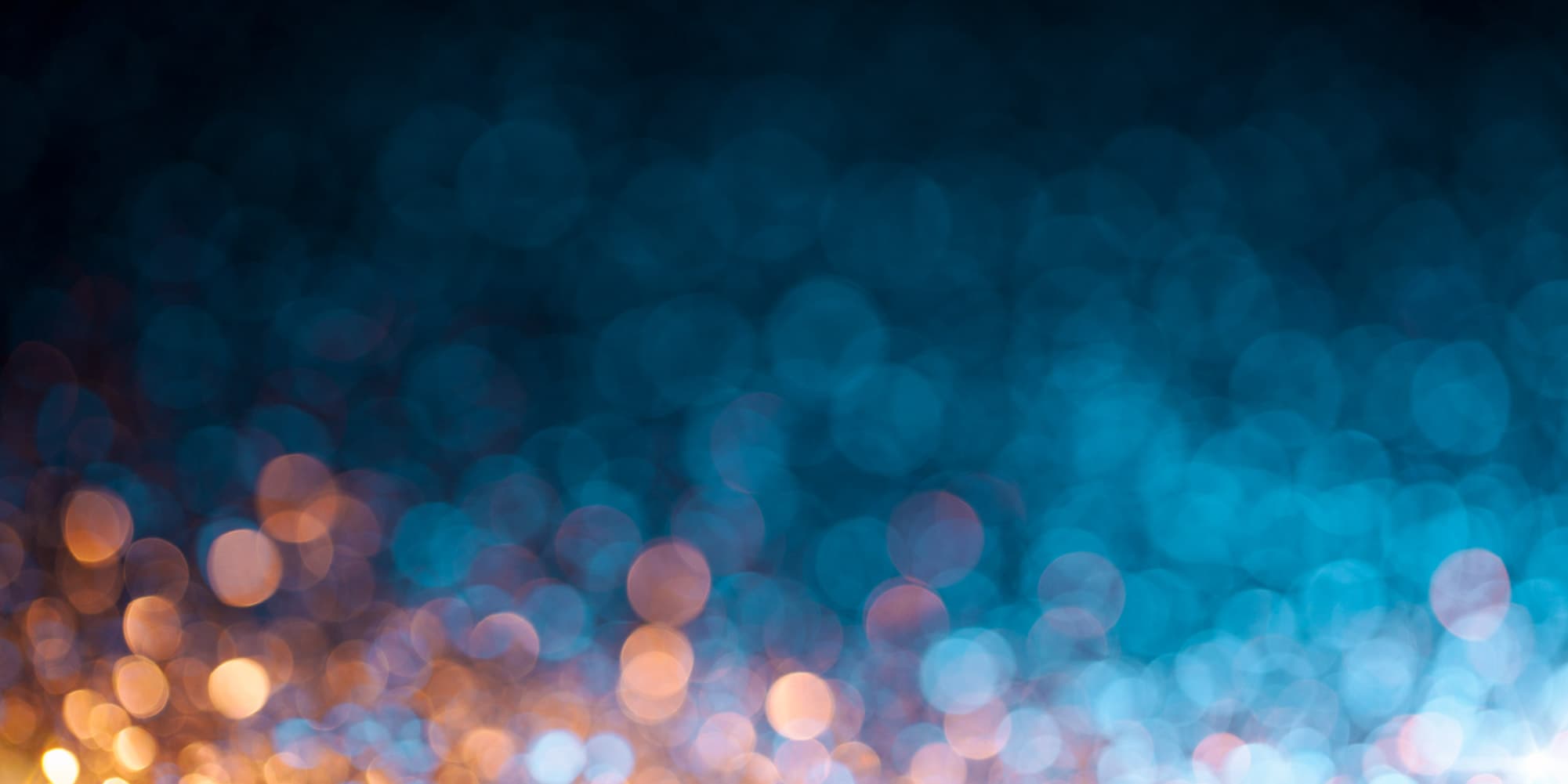
As a result of its holistic approach, the new simulation model is able to deliver much more accurate predictions than other, existing models. The fact that this model also takes account of the cloud cover and wind is another important plus point that other models do not have.
And when you realize that just one cell placed in the shadow produces as much as 80% less energy, then it is also logical that cloud cover should be taken into account in the calculations – even if cloud cover is not a ‘shadow’ as such.
Better than other models
A year after the new simulation model was first announced, it has already been validated in the ‘Oldenburg study’. This is a study conducted at Oldenburg university in which a certain type of solar panels was measured and recorded with precision over a 9-month period. All of the weather parameters (temperature, wind, cloud cover, etc.) were entered into the model and the results were compared with the energy production measured from the panels. The results show that the new model scores better than existing models, specifically with an accuracy rate of 2.8% root-mean-square error (RMSE) for predicting daily production. Other models have a lower accuracy rate of only 3.5% RMSE.
Three practical cases
As we have already said, the simulation model can also be used to test how much a specific modification made to a panel might produce. This means that because it is only a simulation, the cell does not actually have to be manufactured. To illustrate this, three cases were developed, featuring an adjustment using an optical, a thermal and an electrical parameter. This was to demonstrate the importance of the holistic concept.
1. Letting through UV light. Typically, solar cells have a covering film that does not allow UV light to penetrate, because this would damage the film at the backside of the cell. But what would happen if UV light was allowed through at the top anyway? Based on standard models and measurements, making this change would generate an increase in output of 1.8% (under standard lab conditions). But the imec simulation model shows that in real life, this modification would only produce 1% more energy. The model also makes it possible to find out why the actual production of energy is lower. To sum up: the extra light in the cell (UV light) generates heat, which is detrimental for the production of energy.
2. Replacing the film at the back by glass. Traditional solar energy modules are covered with glass on the top and by a ‘backsheet’ at the bottom. However, there is currently a trend towards glass/glass modules. The simulation model has been used to calculate what the differences in energy production are between both types. Different glass thicknesses and backsheet colors have also been tested. To sum up: using glass at the back does not provide a major benefit – and certainly not on an annual basis. However, using a white backsheet instead of a black one creates an increase in energy production in excess of 6%, because photons are also deflected by the white backsheet, thereby returning them to the cell.
3. Comparing cell technologies. Heat has a negative effect on the ability of solar cells to produce electricity: an increase in temperature of 2°C typically causes a 1% reduction in energy production. But not all types of solar cells are equally sensitive to heat. So imagine you had to choose from a variety of different solar cell types that all cost the same: which one would you choose? The simulation model enables you to calculate just how temperature-sensitive the different types of solar cell technologies are. The model was used to test different cells developed by imec, in this instance full BSF, PERC, hybrid SHJ, n-PERT(s), n-PERT(a) and SHJ cells. To sum up: the SHJ cells scored best in terms of temperature stability in real-life conditions (the Oldenburg weather measurements were used, as well as a dataset obtained in collaboration with Kuwait University).
Working together is important
Thanks to models such as this, it becomes feasible in practical terms to use variable energy sources such as solar in everyday life. Having said that, it still remains a very complex issue because there are a great many different players in the energy market. There are also complex regulatory mechanisms in place, which differ from country to country and from continent to continent.
Which is why collaboration is essential. Imec works with the various providers in the energy market, which are very interested in using tools such as this simulation model. In the first instance it can be used to produce better solar cells and modules, as well as to make changes to the way these items are installed and positioned. The model can also used to make micro-grids more stable, while in the longer term it can be applied for the whole electricity network.
Because collaboration is so important, research initiatives such as EnergyVille have come into being, where experts from a range of different fields are able to work together. For example, a test set-up from KU Leuven will be used for the further validation of the simulation model. Imec has great confidence that the EnergyVille collaboration will help achieve green solutions more quickly that will make a difference for everyone in their day-to-day lives.
Want to know more?
- You can ask for the paper entitled “Accurately simulating PV energy production: exploring the impact of module build-up” using this contact form.
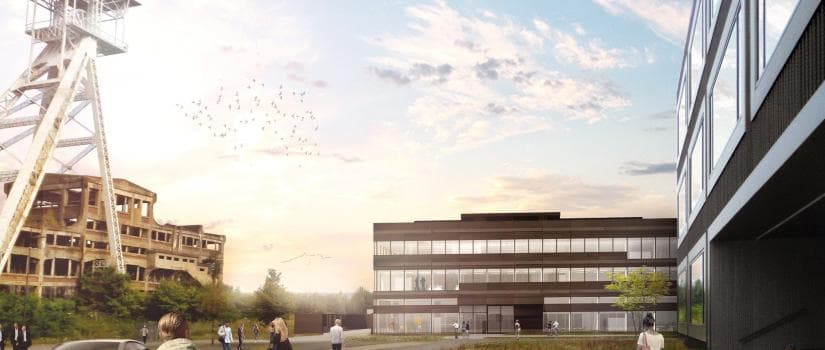
Opening of EnergyVille 2
In the framework of the EnergyVille collaboration, office and lab space is being built at Thor Park, which is part of the former mining site in Waterschei, Belgium. The initiative brings together researchers from KU Leuven, VITO, imec and UHasselt to work on research into sustainable energy and intelligent energy systems.
The second ‘EnergyVille’ building will be opened officially on 31 May 2018. Some 100 researchers from imec and UHasselt will be working at the new facility to develop modules and panels based on thin-film solar cells, as well as a new type of solid-state battery for applications such as electric cars.
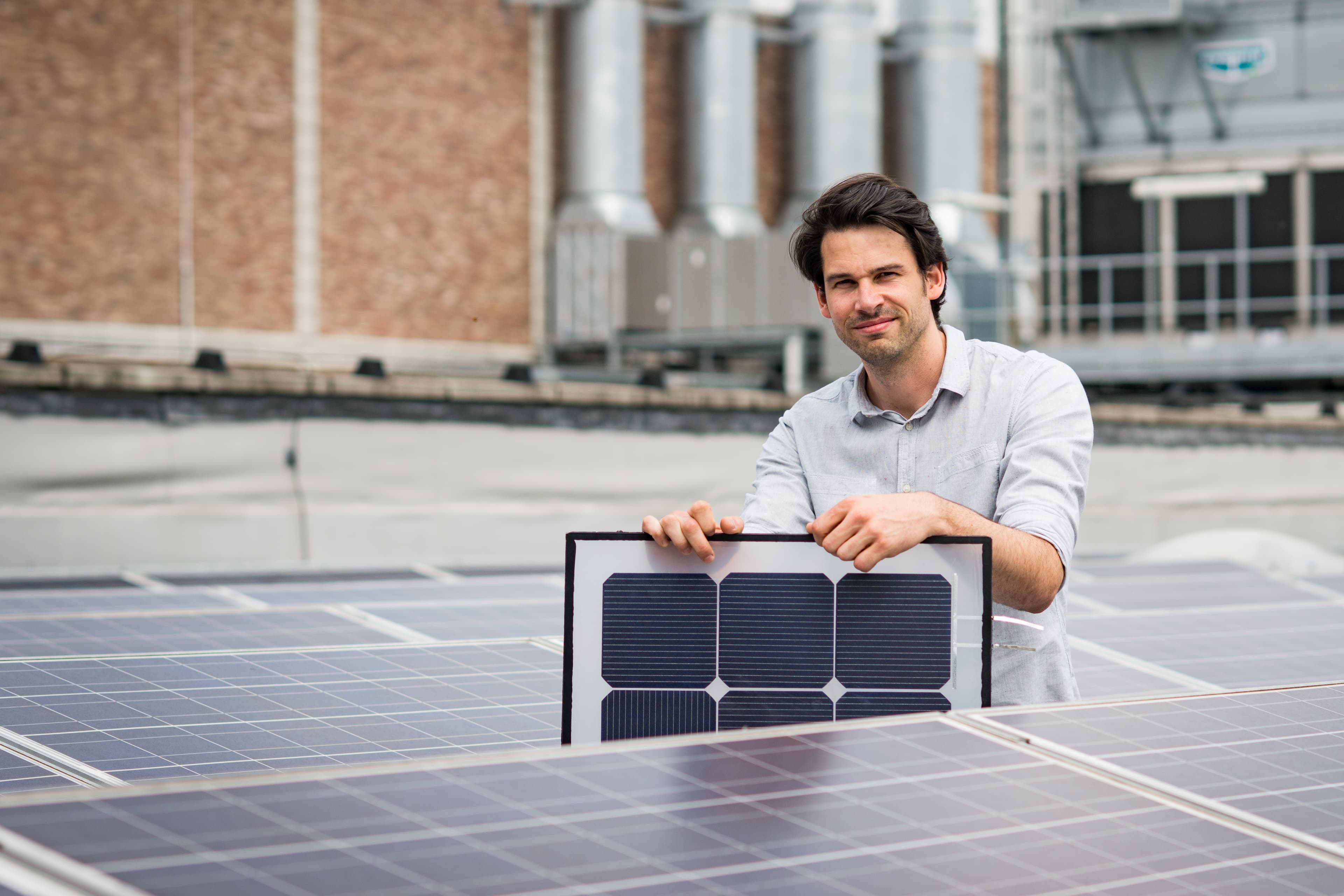
Hans Goverde received his MSc degree in applied physics from the Eindhoven university of technology, the Netherlands, in 2012. The title of his master thesis project was ‘Characterization and optimization of Aluminum Oxide films as p-type Czochralski silicon passivation layer’. In 2016, he obtained his PhD degree (title: increasing and understanding energy yield of c-Si photovoltaic modules) from the KU Leuven in collaboration with the imec photovoltaics group. Currently, he is working as a researcher for the same group at imec.
More about these topics:
Published on:
27 April 2018