To produce a multitude of drugs, foods and beverages, living cells or their components are used in a process called bioprocessing. It involves a complex process flow that needs to be monitored at every step. Today this is mainly done by time-consuming tests, either on-site or in a specialized off-site lab. Can electronic and photonic chips bring the dream of the industry for real-time inline and online monitoring to a reality? Imec researchers present a toolbox of chips that could be used for this purpose: a PCR chip, a micro-ELISA chip, a lens-free camera, an ion sensor, and a mini Raman spectrometer. Silicon chips are ready to revolutionize PAT (Process Analytical Technologies), just as they did with your smartphone!
Olivier Henry, Program Manager Life Sciences and Medical Device Technologies at imec, explains what the main challenges are in bioprocess monitoring and how chip technology can contribute to Industry 4.0 in bioprocessing.
The main challenges in bioprocess monitoring
Both in the upstream and downstream part of the bioprocess, a lot of analysis needs to be done. This to ensure that the growth conditions are perfectly tailored to the cell’s needs (upstream), that there are no contaminants in the end-product and that the product is safe to use and complies with all regulations (downstream).
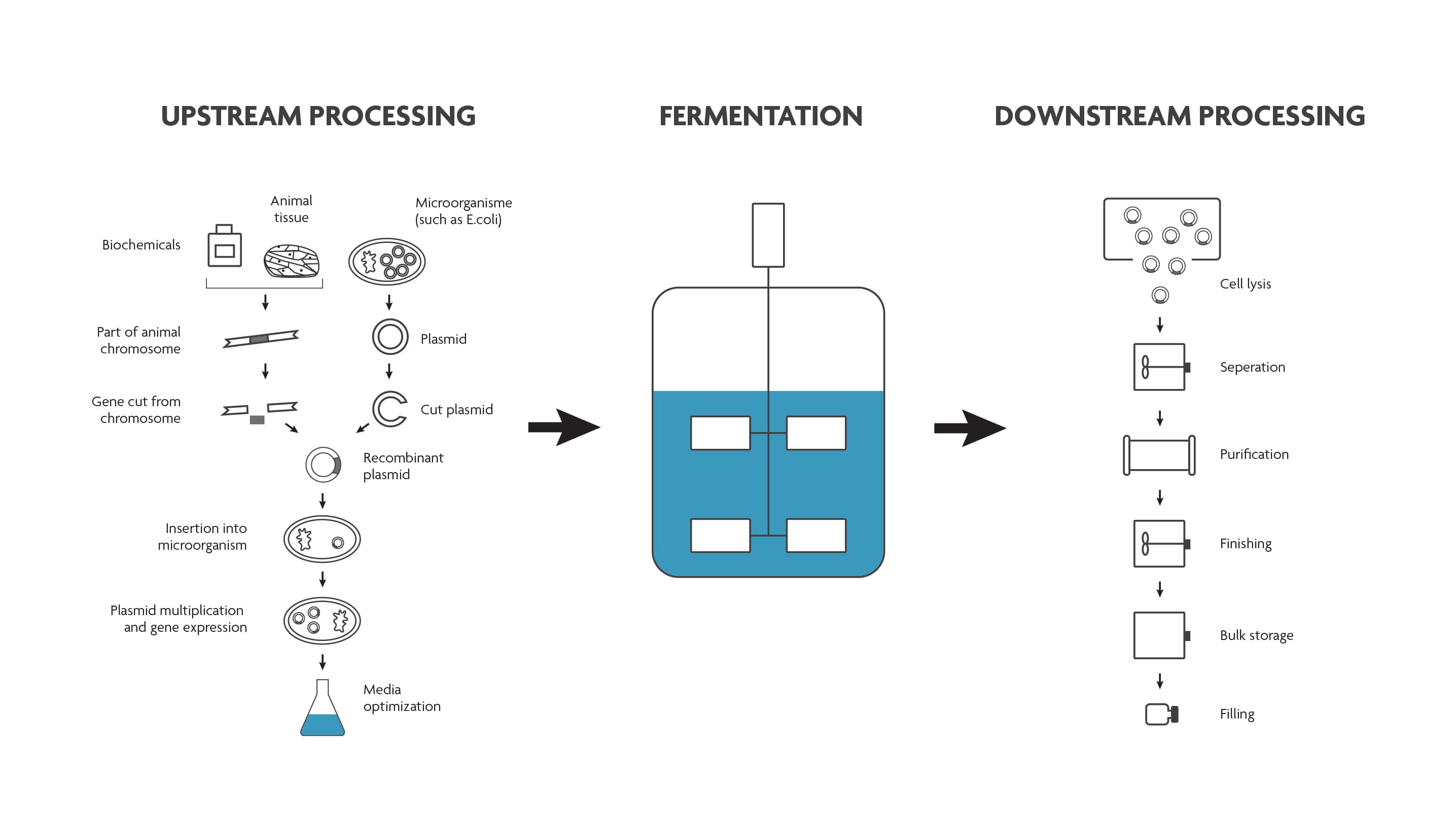
Scheme of a typical bioprocess flow.
Olivier Henry: “Today, a lot of tests (e.g. to ensure safety and sterility) are performed by manually taking a sample out of the process flow. This requires well-trained staff and it poses a risk to the well-controlled environment in which the bioprocess needs to take place. Especially for aseptic bioprocesses (e.g. vaccine production) this is an issue.
Some samples cannot be analyzed on-site and need to be transported to a specialized lab because specific equipment or expertise is needed. In case (part of) viruses or bacteria need to be detected and identified, these tests take a few days. This loss of time makes that the products cannot be real-time batch released.”
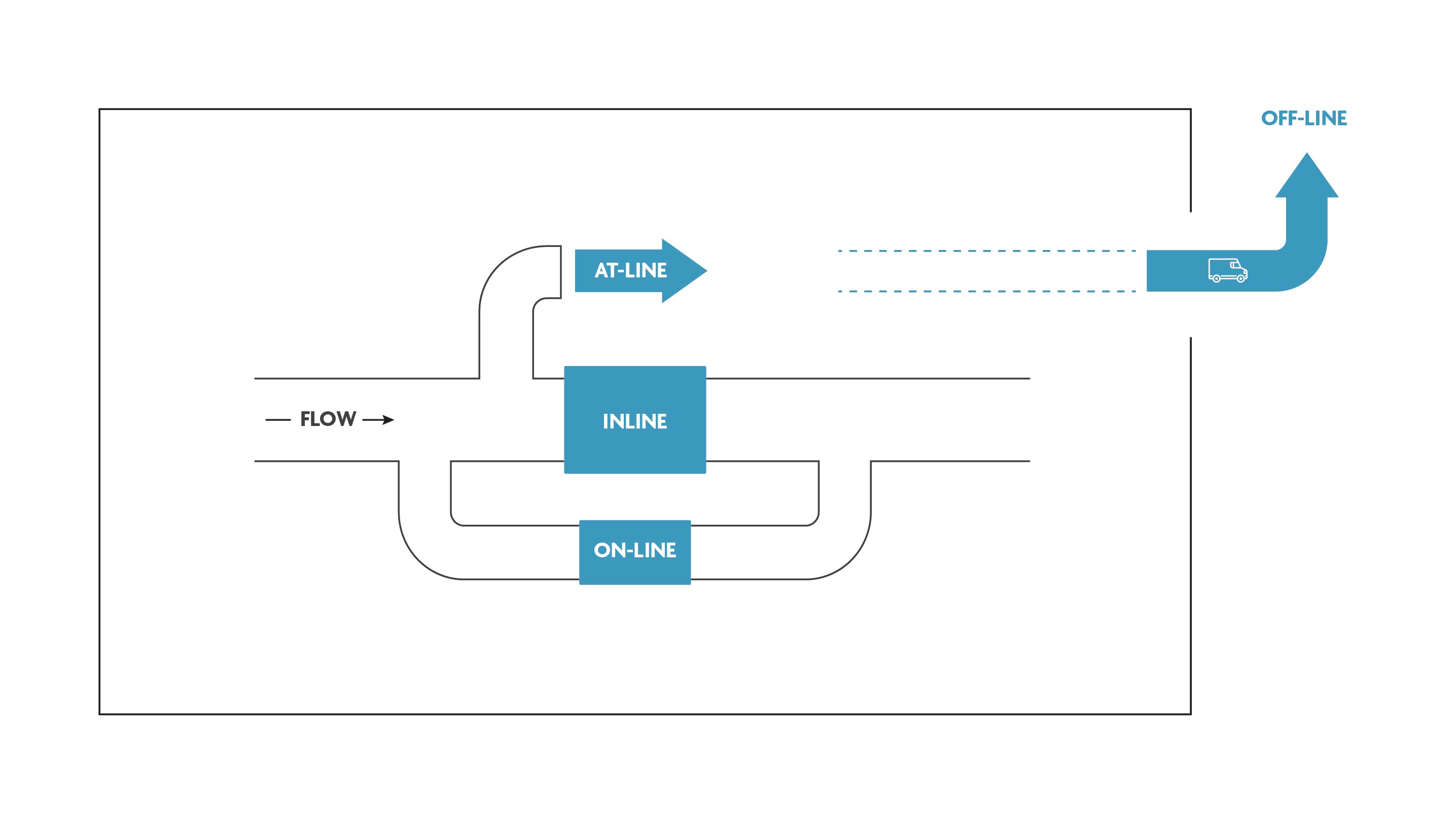
Today, PAT (Process Analytical Technologies) mainly focus on offline testing: samples are taken from the process flow and analyzed on site or in an off-site specialized lab. This is not favourable because of logistics and timing constraints. Chip technology now is mature enough to shift the focus of PAT towards online and inline testing.
Not only because of the process flow there is a time constraint. Just think of cell therapy: the patient needs his/her personalized medication very urgently. Losing time with logistics and testing in off-site labs is not convenient for these types of products.
Olivier Henry: “Another problem with the current PAT and sensors for bioprocess monitoring is fouling: when cells or other compounds attach to the sensor’s surface it is passivated and loses its sensitivity. Therefore, frequent calibration is needed.
And finally, the PAT used require rather expensive equipment so not every bioreactor can be properly equipped or not every process step can be properly monitored.”
Industry 4.0 in bioprocessing thanks to chip technology?
Semiconductor technology, and its products – electronic and photonic chips – have revolutionized the way we work and live. Take for example your smartphone which houses all kinds of functionalities in such a small device.
The same is possible for bioprocess testing: semiconductor technology enables to shrink lab functionalities into small lab-on-chips which even could be integrated into the bioreactors or the process flow. Examples are: mini-versions of PCR reactors, separation modules, cell sorters, spectroscopes and microscopes, ... and all this at a low cost because semiconductor technology focuses on mass production and parallelization.
Olivier Henry: “Furthermore, semiconductor technology uses highly controlled fabrication processes that produce devices with very tight specifications and reproducible performances. This in turn allows to integrate different sensing modalities onto a single chip in a very compact footprint (e.g. optical, acoustic, electrical), create arrays of sensors to multiplex measurements, integrate fluidics for sample delivery, and opens new possibilities for sensor calibration and continuous operation.”
A toolbox of chips
How can chips contribute to a real-time, calibration-free, fast, remotely operated and inline bioprocess monitoring? Olivier Henry: “The examples below demonstrate the capabilities of chips for a multitude of applications in the life sciences, measuring physical, chemical and biochemical parameters. By further developing and tuning these technologies, it is a small step to further advance PAT for bioprocessing.”
A miniaturized multi-ion sensor
Imec researchers developed multi-ion sensors for fluid monitoring. They use the same technology – ion-sensitive electrodes – as today’s large, expensive one-target sensors, but by applying its expertise in chip technology, imec was able to make the system much smaller. In fact, a range of electrodes can be placed on an area of about 1cm². Olivier Henry: “Next to measuring specific ions (Cl-, Na+, K+, Ca2+, and NO3-), it can also measure the pH, dissolved oxygen and salt concentration of a solution. It is a generic platform which can be tailored towards specific applications: by changing the selective membranes on the electrodes, the sensor can be adopted to detect other ions. It is also relatively simple to integrate e.g. a temperature sensor onto the same chip.
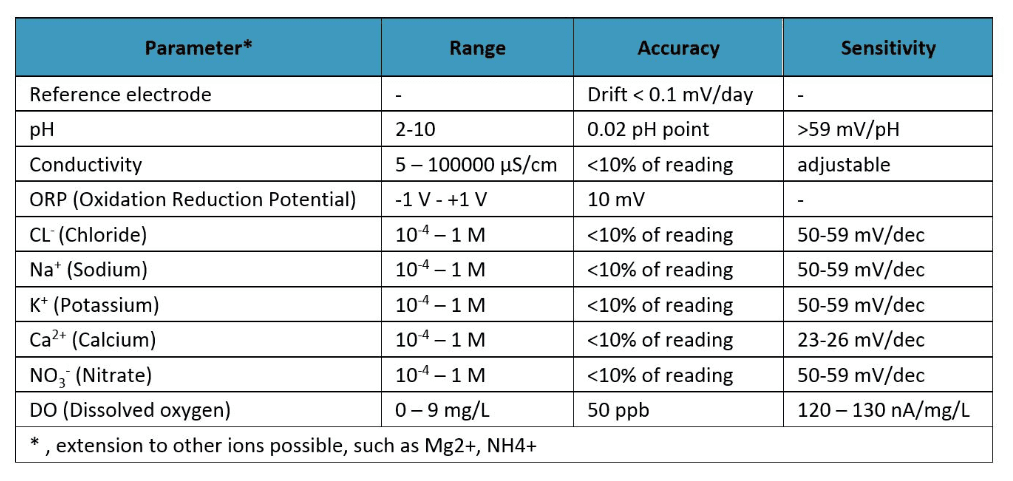
Properties of imec’s fluid sensor and some of the ions that have been measured with it.
An essential component is the reference electrode, which is also incorporated into this small area. Also, the packaged sensor has readout electronics and a radio device for transmitting the data wirelessly. So, in fact, it is a sort of miniature box of building blocks to which you can add the bricks that you need. And because the system is based on chip technology, it is all very compact and inexpensive.”
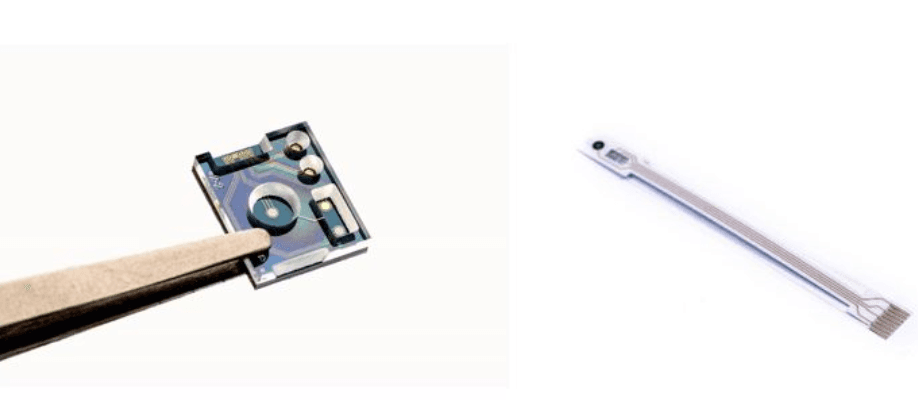
Integrated pH and ion sensors in a microfluidic device. There are two versions of this ‘fluid sensor’: one on silicon and the other on a bendable substrate.
This kind of miniaturized fluid sensor could be used in the upstream processing for yoghurt, beer, wine and cell therapy production. Because of its low-cost properties, the technology also becomes available for e.g. small breweries for keeping a constant, real-time eye on the composition of the solution in the bioreactor. It can also definitely make a difference for cell therapy, because there you are using a material that varies a great deal – the cells of a specific patient – which may react very differently to the same culture conditions.
A mini-Raman spectroscope
Raman spectroscopy is used to identify materials – fluids, powders, solids – and to learn more about the materials composition. By shining laser light on the material and analyzing a specific part of the scattered light, a spectral chart can be made. In this, one can recognize the spectral ‘fingerprint’ of each material and compound.
A well-known spectrometer is based on the Michelson interferometer. The disadvantage of this design – particularly if you want to miniaturize it – is that two mirrors are used, one of which moves.
Imec researchers are developing an integrated photonics version of such a scheme, without moving parts: the integrated spatially heterodyne spectrometer, a patented solution for a miniature Raman spectroscope.
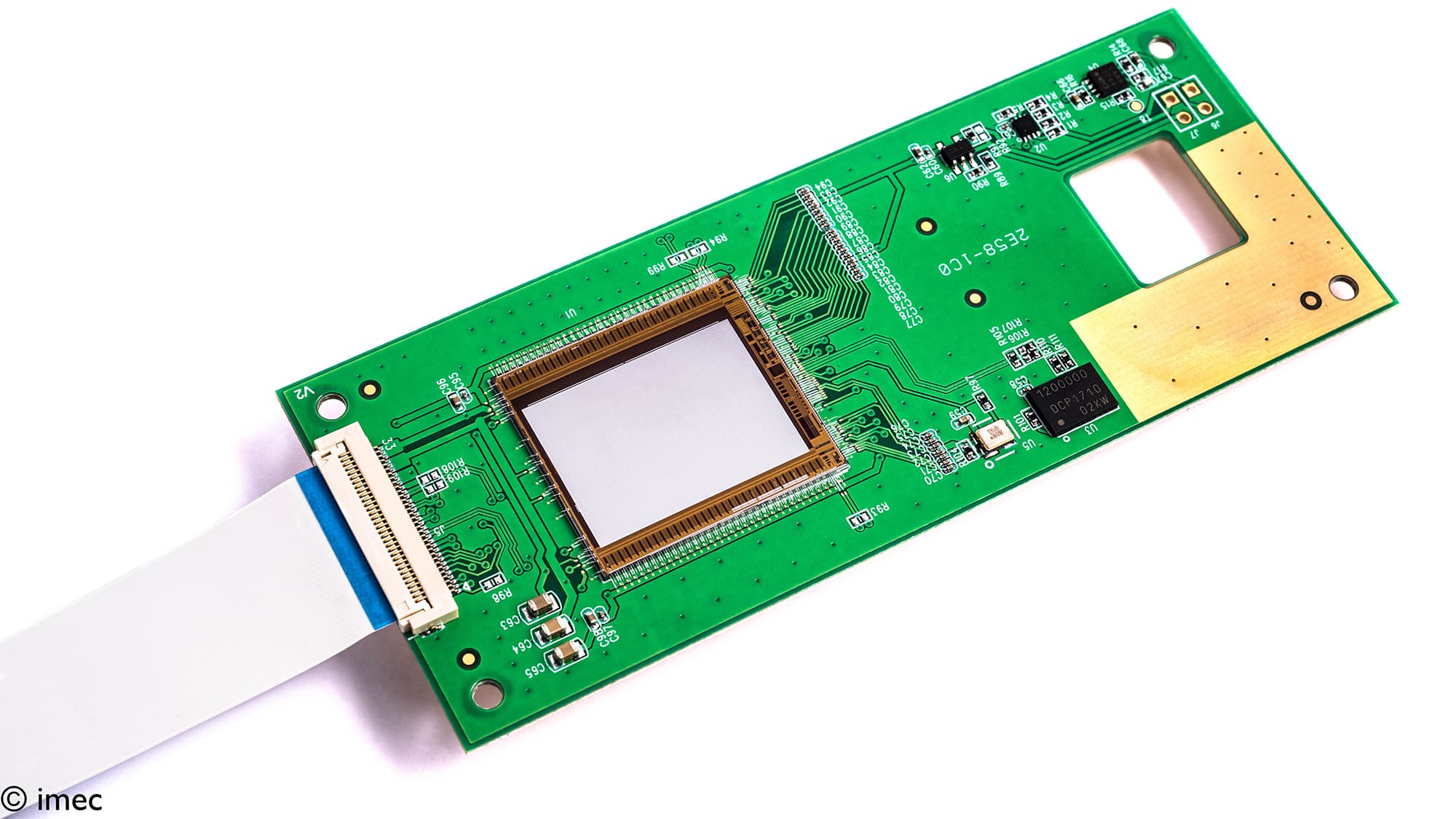
Imec’s on-chip Raman spectroscopy solution, using a million interferometers on top of a CMOS image sensor. This build-up allows for extreme miniaturization and, using chip technology, the price of the device can be much lower than current devices.
Olivier Henry: “Raman spectroscopy is useful in both the upstream and the downstream bioprocess, to monitor the composition of the fluids. For example, to decide if the level of nutrition is sufficient for a certain cell culture to maintain their growth by following analyte consumption (e.g. glucose) and metabolites production (e.g. lactate) and potentially enable feedback-controlled bioprocess optimization.”
A lens-free microscope
For some process steps (e.g. in cell therapy bioprocessing) it is important to have a visual inspection of the cells’ morphology to get direct feedback on the viability and overall condition of the cells. Normally this is performed by a process operator by taking a sample out of the bioreactor for inspection with a microscope. This manual observation holds significant limitations, for example the fact that certain characteristics are almost impossible to correctly quantify manually. This evaluation also typically suffers from operator-to-operator variability.
Olivier Henry: “Imec has developed a lens-free imaging cytometer technology that can be integrated with bioreactors and could offer a solution for in-flow cell imaging. The lens-free system uses a coherent light source (e.g. LED or LASER) and a CMOS imager to capture the light that is diffracted off small objects. The captured diffraction pattern (called a hologram) resembles the ripple effect pattern caused by an object landing on a water surface. Custom software algorithms are required to reconstruct the hologram into an in-focus image that resembles the object that is being imaged. It is a compact solution, which enables a large field of view and very good resolution, at an affordable price. A machine-learning based image analysis and classification pipeline is also being developed to evaluate the images and differentiate between specific cell types using powerful classification algorithms.”
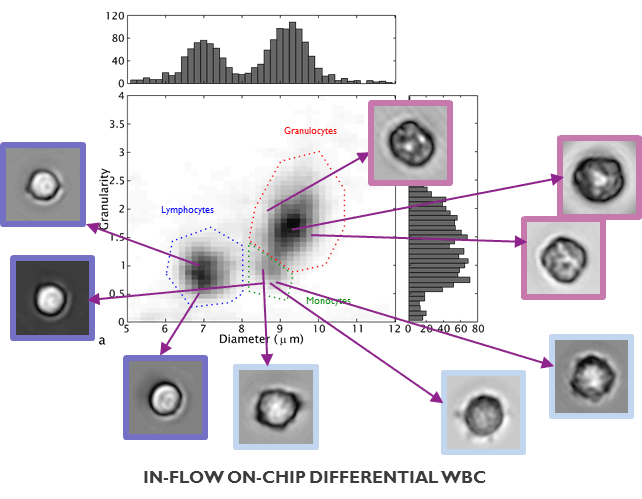
Imec’s lens-free imaging technology enables the direct cell inspection and classification either in static culture but also at a sufficiently high frame rate to be operated in-flow. To demonstrate its capabilities, a mixed white blood cell sample was investigated. The lens-free imaging technology was capable of differentiating three cell types in the sample: lymphocytes, granulocytes and monocytes.
A prototype of the lens-free inline holographic microscopy device for static cultures.
The lens-free imaging technique was already used in a conventional cell culture incubator common to cell biology laboratories, for monitoring and evaluating in vitro cardiac contractility. The advantage in this case was a 50-fold larger area with 50-fold less expensive equipment. Other set-ups, based on the same principle, were developed for single-cell migration assays to compare the migratory potentials of metastatic and non-metastatic breast adenocarcinoma cells. Also in this case, the compact set-up was housed in a standard cell culture incubator.
Finally, the lens-free microscope was integrated in a lab-on-chip device that was developed to sort cells. Tens to hundreds of these cell-sorter devices – only 1 mm² in size - can be combined on a chip to enable high-performance cell sorting.
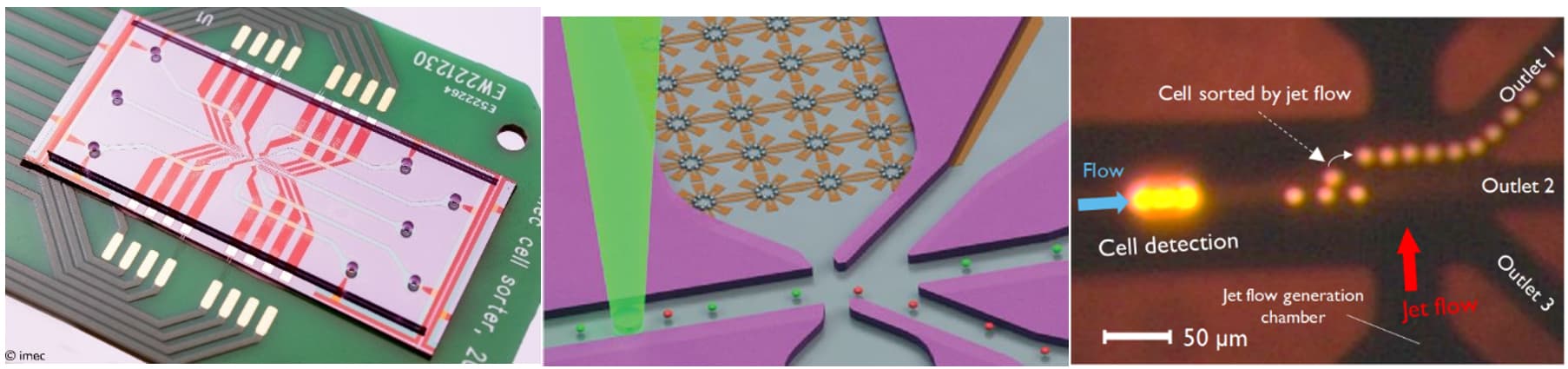
Imec’s cell sorter technology that makes use of lens-free microscopy.
A protein-detection chip
By analyzing by-products of cell metabolism – proteins and enzymes – either being taken-up from or secreted into the culture medium, the biological state of cells can be determined. Current technologies e.g. based on immunoassays, mass spectrometry, chromatography or spectroscopy are not always available in on-line setups, require large efforts in sample preparation and often lack specificity in complex culture media with a high protein background.
The mainstream approach for protein sensing is the use of immunoassays, mostly ELISA in one of its many variants. It is a label-based approach in which the label is responsible for signal increase and the suppression of background events and thus for the good sensitivity and specificity of ELISAs. A drawback is that any unbound labels need to be washed away before the measurement can be performed, complicating the overall workflow and increasing the time to result. As an alternative, many label-free techniques have been explored, but these invariably suffer from poor specificity and, hence, have poor limits of detection in complex matrices.
Olivier Henry: “As an alternative, imec develops photonic-based immunoassays. Photonics is a well-known technology used in e.g. glass fibers to transport data in a more efficient way. But photonic waveguides and other components can also be used for life science applications. To enable this, imec – together with partners in the European PIX4life project10 – has set up a silicon nitride – for visible light – photonics pilot line. A full library of photonic components is available for life science companies to build the next generation of photonic biochips. With this photonics platform – and more specifically with the use of photonic waveguides – imec has built a fluorescent immunoassay which could be used to monitor relevant proteins in bioprocessing.
Its specificity, sensitivity and detection limits are comparable to ELISA, but it allows a rapid kinetic, wash-free read-out. The key element of this immunoassay is a photonic chip which could be easily integrated in a bioreactor. Note that this technique is generic and that any protein of interest can be detected with this immunoassay.”
A DNA amplification & detection chip
PCR or polymerase chain reaction is a well-known technique to do DNA or RNA detection in samples. Olivier Henry: “Imagine all lab functionalities needed for PCR, being shrunk and placed on a chip. In the case of bioprocessing, this could be interesting to test for contaminants such as bacteria or viruses, in a compact, automated and fast way, very suitable for a PAT.”
Imec developed a small silicon chip that can perform sample preparation, DNA extraction and quantification and include small reaction chambers to carry out a full PCR reaction within a few minutes. The chip contains an etched 1.3 µL meandering microreactor, integrated aluminum heaters, thermal insulation trenches and microfluidic channels and has been applied to the rapid and sensitive detection of viruses and bacteria in clinical samples.
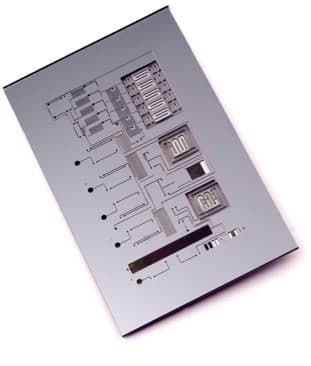
A silicon-based chip (1x2cm) to perform sample preparation, DNA extraction and quantification, with qPCR reaction microchambers. Each reaction chamber is enclosed by insulating trenches.
Conclusion
Olivier Henry: “We are convinced that chip technology can revolutionize bioprocess monitoring and allow for real-time inline and online monitoring and, as a consequence, potentially enable real time batch release in the future. In this article, we showed five different technology platforms – the multi-ion senor, the Raman spectroscope, the lens-free microscope, the protein-detection chip and the DNA amplification & detection chip - that can be used at different stages of the bioprocess. Although these technologies are not yet tuned to the specific needs of bioprocess monitoring – we only showed examples of projects in the life science field – it is feasible to deploy each of the technologies as PAT for inline and online monitoring. Together with companies active in the field of bioprocessing, we want to further develop tailored solutions and help implementing the concept of industry 4.0 in the field of bioprocessing.”
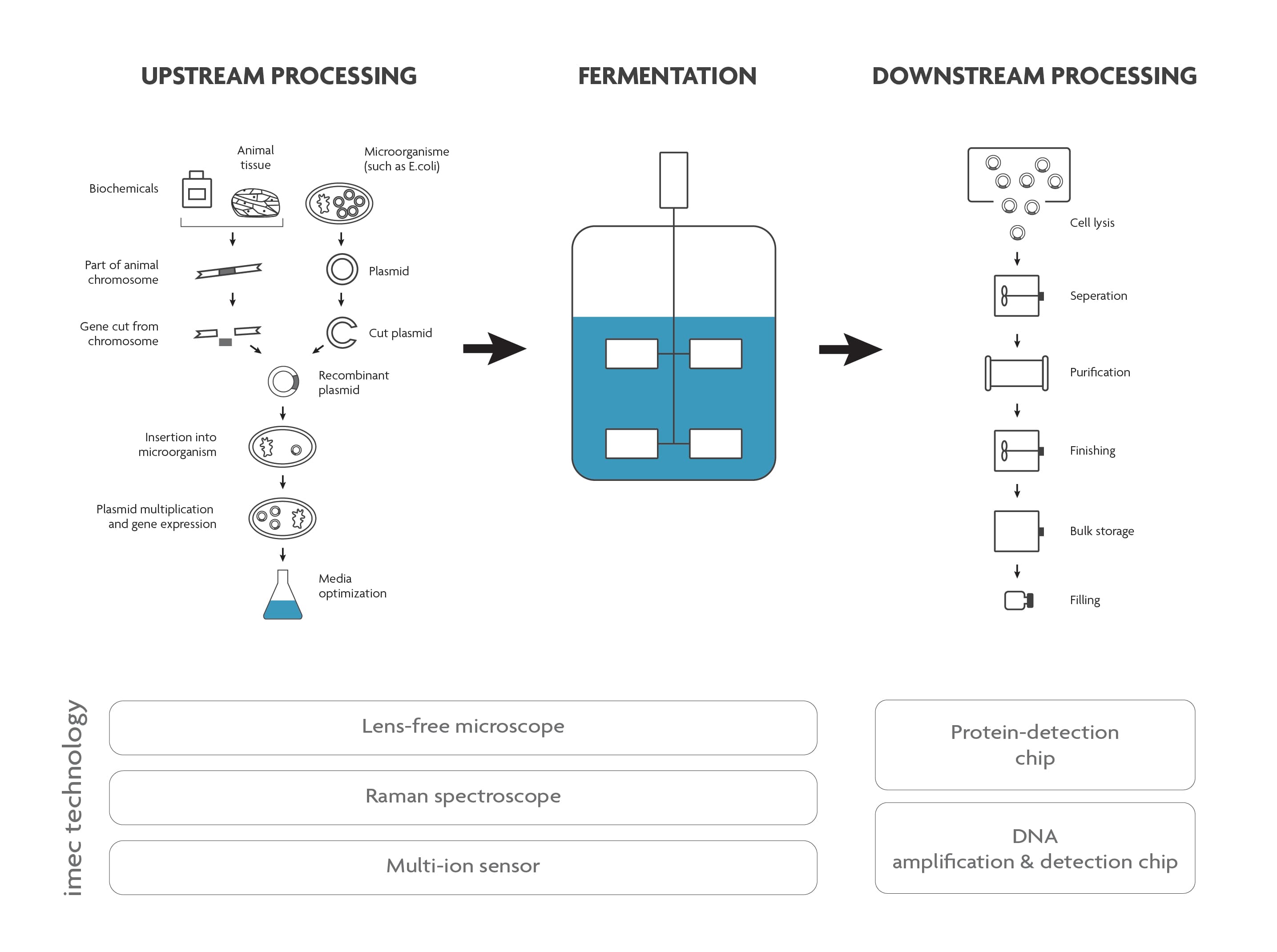
Want to know more?
Are you interested in working with imec on one of these technologies for bioprocess monitoring, please let us know.
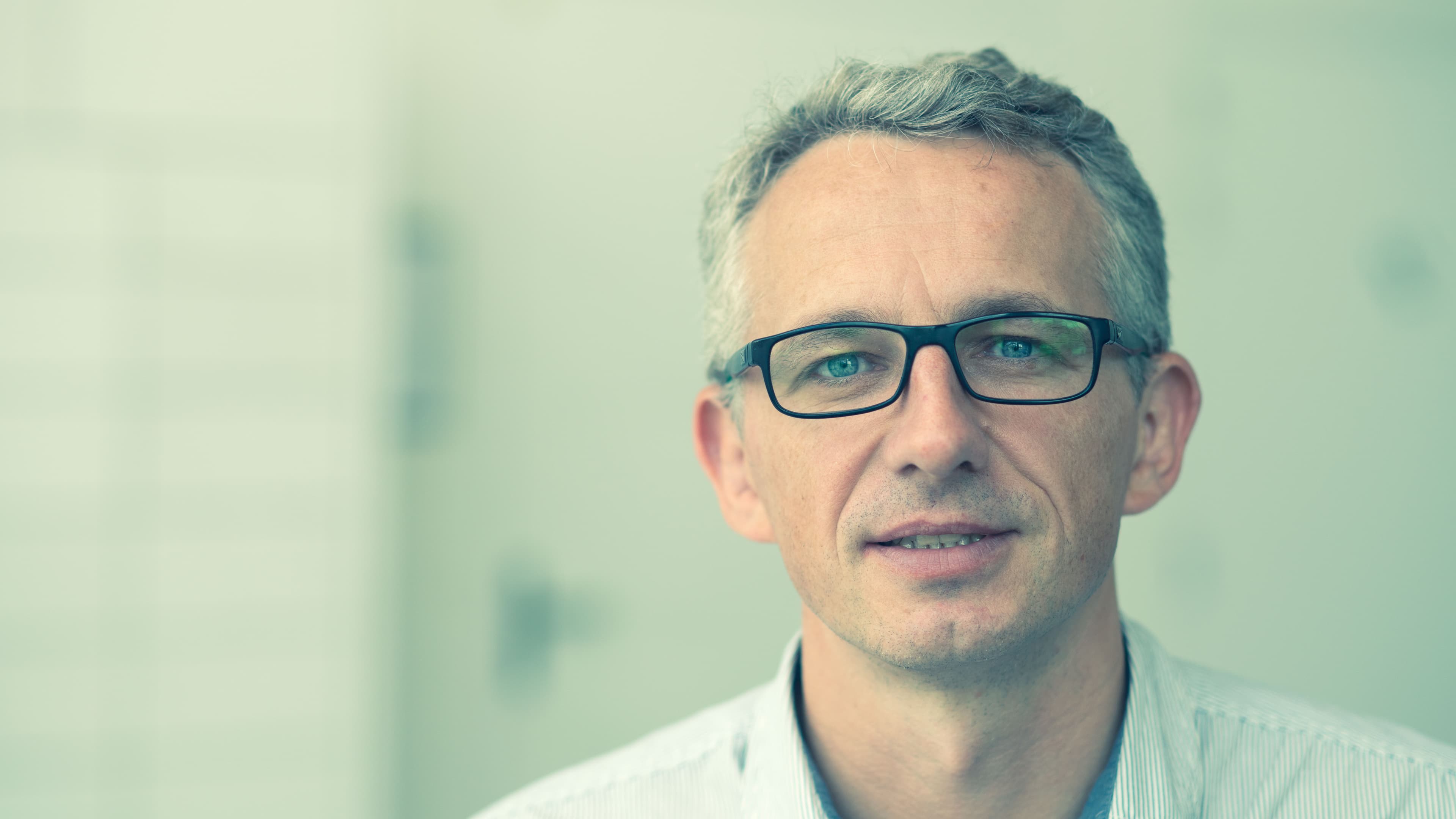
Dr. Olivier Henry research interests and expertise lie in the development of new integrated microdevices with application in diagnostics, chemical and biochemical synthesis and cell and tissue microengineering. With a background in applied chemistry and instrumentation, he holds a PhD in Biomedical Science (Cranfield University, 2005). After holding research positions at GSK, Sphere Medical and the Universitat Rogira i Virgili, Olivier worked as a Senior Staff Engineer within the Advanced Technology Team at the Wyss Institute for Biologically Inspired Engineering at Harvard University, heading the Microfabrication and Sensors team and developing new approaches for the monitoring of microfluidic-based cell cultures, medical diagnostics and information storage technology. He joined imec as Program Manager for Life Science & Medical Device Technologies in 2018 where he bridges multiple technology disciplines to support and define technological roadmaps, support imec’s business development and relations with customers eager to build the next generation chips required to advance life science technology. He has co-authored over 40 peer-reviewed publications and hold several patents in the field of polymer and electrochemistry, microfluidics, sensing and diagnostics.
More about these topics:
Published on:
8 September 2019